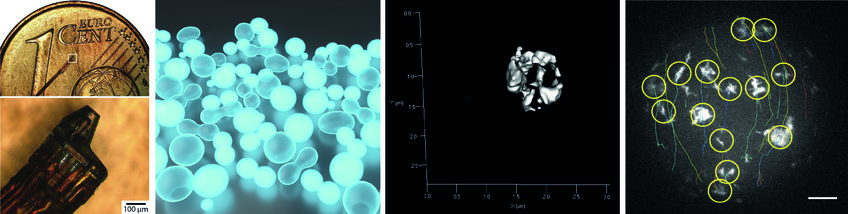
Research
Microfluidics, cell free protein synthesis, microcompartments, MinCDE pattern formation, bacterial division, archaeal division, Z-ring, actomyosin
The specific responsibilities of the Schwille Group within the MaxSynBio consortium includes:
Microfluidics and methods for compartment creation
Schwille group will further improve the microfluidic phase chip technology implemented to dynamically and reversibly probe liquid-liquid and liquid-solid phase transitions across reversible composition and/or temperature gradients at high throughput.
Efficient and customized cell free protein synthesis (Protein Facility Martinsried)
Schwille group develops a versatile technology platform, which can be easily transferred to and adapted by the collaborating groups. Support in establishing cell free protein production through standardized modules. These will include a hydrophobic environment optimization for membrane proteins, and circuitry for selected stoichiometric production of multiple interacting proteins, including complexes.
Transfer of cell-free protein production into microcompartments
Cell-free protein synthesis will be combined with the microfluidic droplet and vesicle production with collaborating groups. In corporation of membrane proteins will be accomplished in combining the cell-free protein expressions systems with membrane insertion or attaching factors.
Minimal protein modules for compartment geometry sensing and polarity induction
The MinCDE protein system is a paradigm for pattern formation and polarity induction based on a minimal set of functional modules. Our aim is to elucidate the key motifs that could be transferred to other protein or polymer systems, for the design of generic set of molecules to sense compartment geometry and establish spatial cues for downstream processes, including division. We will continue to address key design features of the Min system, such as cooperativity of membrane binding, the actual feedback mechanisms based on structural switches, and the role of membrane structure and composition.
Bacterial and Archaeal division rings
One of the most prominent features in bacterial cell division, being supposedly among the simplest in single-cell organisms, is the so-called contractile Z ring. Its primary protein constituent, FtsZ, is a tubulin homologue with remarkable self-assembly and self-organization properties. Work by us and other groups has revealed that FtsZ can form dynamic rings in the range of few microns that are able to deform membranes, but whose role in the actual constriction remains to be revealed.
In order to construct larger contractile rings of many micrometers able to deform and divide GUVs, we aimto extend our work on a fascinating crenarchaealdivision machinery, ESCRT-III-like paralogs that have been claimed essential for cell division in Sulfolobus acidocaldarius. In particular, we will investigate in a time-resolved manner the interactions of CdvA, CdvB, and DNA to lipid bilayers via SLB or GUV membrane models.
Spatially confined active contractility modules based on actomyosin
This task will build on our earlier work of locally contractile minimal actomyosin cortices on model Membranes. In this project phase, the most important task is to restrict the surface coverage of vesicles or droplets by actomyosin to contractile zones, ideally ring-shaped and with small lateral extension. Intrinsic self-organized confinement may be established using the Cdc42-based symmetry-breaking module from the first funding phase to induce polarized growth of actin networks. Alternatively, a positioning system based on key functional motifs of the MinCDE system can be explored. Lastly, we will establish photoactivable anchors in order to target a contractile zone by confined light illumination.